Background
Critical nutrient levels are presented here to provide some interpretation of canola soil and tissue tests for both macro nutrients and micro nutrients.
A ‘traffic light’ format has been used with red indicating greater than 25% yield loss is likely, orange indicating between 15-25% yield loss, yellow between 5-15% yield loss and green less than 5% yield loss. Canola growers can use this information to easily determine the urgency of addressing a particular nutrient issue or soil constraint.
These critical values can be used as a guide to the approximate levels of yield loss expected, for macronutrients and trace elements. They are necessarily simplified and generalised and are not intended or able to replace professional assessment and recommendations. A Quick reference guide (tables only version) is available from the right hand menu under Documents.
To view images of nutrient deficiency in canola, or view information about other constraints in canola, see DPIRD's MyCrop canola diagnostic tool.
Nutrient removal in canola seed and stubble
Harvesting seed in any rotation is exporting nutrients from the farming system so it is important that the nutrient balance in a rotation is maintained for sustainability.
Canola is a valuable crop for the farming systems in Western Australia. It is an ideal break crop for cereals, provides good weed control, lengthens cropping rotations and can be a profitable cash crop. Growers need to be aware of the nutrient concentrations in seed and stubble when planning their cropping and fertiliser program.
Plant available nutrient in the seed and stubble
Canola is considered to be very efficient at extracting nutrients from the soil.
Nutrient concentrations in canola stubble and seed vary greatly depending on season, management practices, available soil nutrient levels and variety. The ranges of the amount of nutrients commonly found in canola seed and stubble in WA crops are shown in Table 1.
Macronutrient content (kg/tonne) | Macronutrient content (g/tonne) | ||||||||
N | P | K | S | Mg | Ca | Cu | Zn | Mn | |
Seed | 26-40 | 2-6 | 6-9 | 3.2-6 | 2-4 | 2-4 | 1.5-5 | 22-45 | 16-40 |
Average | 33 | 4 | 7 | 4 | 2.5 | 2.5 | 2.1 | 30 | 27 |
Stubble | 6-10 | 1-3 | 18-30 | 3.4-6.5 | 0.6-2 | 4-7 | 2-6 | 10-15 | 10-30 |
Windrowing redistributes nutrients
There is little removal of canola stubble from the cropped paddock. However, important quantities of nutrients can be redistributed into windrows that would not otherwise have occurred in direct harvesting methods. The windrowing and burning of stubble causes little or no direct loss of plant-available nutrients, except for sulfur which may be lost as sulfur dioxide. After burning the nutrients are redistributed and return to the soil in the ash after burning. This is particularly true for potassium with higher soil potassium levels sometimes found under windrowed and burnt canola stubble.
Losses of nutrients, other than in the seed
To maintain the level of plant-available nutrients in the soil in successive canola cropping systems, more nutrients must be applied than is removed in canola seed. This is to compensate for losses, other than in crop products. The nature and extent of other losses vary between nutrients, soil properties and the amount and seasonal distribution or rainfall.
Loss of nitrogen
Nitrogen fertiliser is most prone to losses by biological processes and leaching in WA. Commonly the amount of nitrogen fertiliser needed to maintain soil fertility is about double the amount of nitrogen removed in seed. As there is about 50-70% efficiency in plant uptake and redistribution of nitrogen to seed, the use of nitrogen content in seed usually underestimates the amount of nitrogen fertiliser needed to be applied.
In rotations of canola with legumes, the legumes may add sufficient nitrogen to the soil for that removed in crop products.
Loss of phosphorus
Phosphorus is most prone to losses caused by chemical reactions (known as fixation, sorption, or retention) in the soil. Losses of phosphorus by fixation in WA soils are in the range of 2-10kg of phosphorus per hectare per year, with about 4kg/ha phosphorus being most common.
Growing crops with high analysis fertilisers such as DAP (di-ammonium phosphate), with a low sulfur concentration, may result in a decline of soil sulfur supply for the cropping production system. Periodic application of superphosphate is one strategy to replace the calcium and sulphur removed in crop products.
Loss of sulfur
Sulfur in the sulfate form is easily leached in sandy soils and on sandy soils in WA. As little as 15% of applied sulfur can remain in the top 50cm of soil one year after application. This can result in sulfur deficiency and is more likely to occur in higher rainfall areas or during wet seasons. Loss of sulfate through leaching is less likely on soils with higher clay content at depth (including duplex soils) than on deep sands. This is because sulfate leached from the top soil remains in the clay containing subsoil and becomes available to the crop once its roots systems develop.
Canola crops are generally seeded with a sulfur containing compound fertiliser that supplies enough phosphorus for the entire crop, but only a starter amount of nitrogen and sulfur. Additional nitrogen and sulfur are then applied post emergence.
Loss of potassium
Potassium is prone to leaching on soils with low clay concentrations and low organic matter, especially in high rainfall areas. Only on very sandy soils in the wetter parts of the grain belt are potassium losses by leaching likely to be significant. Many of our sandy soils have low reserves of plant-available potassium. However, soils of the Great Southern and some duplex soils are now found to be deficient in potassium for cropping. Deeper soil testing provides a more complete picture of potassium availability in a paddock and distribution within the soil profile.
Potassium concentration in the stubble of canola is much higher than that found in the seed. There will be a greater proportion of potassium redistributed into the windrows than for other nutrients. Consequently, the need to replace potassium removed in crop products will become increasingly common where the stubble has been windrowed and burnt. Where the stubble has been burnt, the in-between area has often been diagnosed as potassium deficient after swathed and windrowed crops. Potassium problems in wheat following canola have been noted in paddocks where the potassium level in the top 10cm of soil have been less than about 80ppm.
Loss of calcium and magnesium
Most WA soils have large reserves of plant-available magnesium and calcium, however calcium deficiency does occasionally occur in canola; presenting as malformation at flowering and pod formation (known as tipple topple). Magnesium deficiency has not yet been observed in WA canola crops.
Loss of micronutrients
The amounts of micronutrients (copper, zinc, manganese and molybdenum) removed in canola seed have a negligible effect on their supply, in plant-available form, in soils. The need for application or re-application of the micronutrients are almost entirely governed by soil processes other than product removal, such as soil acidity and fixation by soil materials.
Macronutrients in canola
Phosphorus
Interpreting soil and tissue tests
Yield loss | >25% | 15-25% | 5-15% | <5% |
Phosphorous 0-10cm soil test (mg/kg)(Colwell P) | <13 | 13-16 | 16-22 | >22 |
Phosphorous in whole tops at seedling stage (%) | <0.27 | 0.27-0.35 | 0.35-0.4 | >0.4 |
Phosphorous in whole tops at rosette stage (%) | <0.18 | 0.18-0.23 | 0.23-0.27 | >0.27 |
- Critical levels can be affected by pH, with less phosphorus available at pH below 4.8 and above 7.5
- Critical soil extractable phosphorus levels are affected by the phosphorus buffering index (PBI). Higher PBI soils require more phosphorus to be applied due to the strong adsorption of phosphorus on soil particles. Phosphorus soil decision tools consider PBI within the decision making system.
- High rates of phosphorus fertiliser near the seed can cause toxicity and germination problems, so higher rates are best banded away from the seed.
- Generally it is not effective or economic to correct phosphorous deficiency during growing season.
Phosphorus in soils and plants
WA Wheatbelt soils were naturally low in phosphorus before clearing for agriculture. Continual use of fertiliser has built up levels of phosphorous in wheatbelt soils, however canola yield losses caused by phosphorus deficiency are still common.
Plant requirement for phosphorus is particularly high during the early stages of growth. Phosphorus supplied by the seed is depleted very rapidly, after which seedlings are dependent on the developing root system to maintain supply from the soil.
Early phosphorus deficiency is often transitory while the root system is developing and is compounded by dry soil soon after the emergence of canola seedlings. Often deficiency symptoms disappear as the root system develops or when topsoil re-wets following rainfall. Ensuring the developing seedling can access sufficient phosphorus is important because even transitory deficiency can result in lost yield.
Phosphorus availability in soil
Most phosphorus in fertiliser is water-soluble; however phosphorus is highly immobile within soils and reacts with other elements in the soil solution (precipitation) and on the surface of soil particles (adsorption). These reactions reduce the amount of soluble phosphorus available for root uptake by growing crops.
The ability of a particular soil to react and adsorb soluble phosphorus is measured as the phosphorus buffering index (PBI). For soils with the same level of extractable phosphorus (Colwell P), deficiency is most likely to occur on soils with high PBI, such as Darling Range forest gravels, compared to sand (low PBI).
As a guide, soils considered to have low-moderate phosphorus buffering index have a PBI less than 8. Soils consider to have medium buffereing index have a PBI between 9 and 15. Soils considered to have high to very high phosphorus buffering index have a PBI above 16.
In soils with a long history of phosphorus fertiliser, phosphorus adsorption sites gradually become saturated meaning a higher proportion of applied fertiliser remains in solution and available to developing crops for longer. Phosphorous adsorbed to soil particles can also return to the soil solution and become available to following crops, meaning it has good residual value.
Phosphorus availability is also linked to soil pH, with solubility decreasing in both acidic and alkaline soils. The ideal soil pH range for canola is between pH 5.5-7.5 and phosphorus availability is high within this range. If soil pH is outside of this range, phosphorus solubility decreases, reducing availability to plants.
Growing canola on soils with a pH outside this ideal range can have other consequences related to phosphorus nutrition. Canola is particularly sensitive to aluminium toxicity, a common problem on wheatbelt soils with pH below 4.8. This can result in root pruning and reduced root exploration of the soil which can markedly increase phosphorus deficiency. Application of lime to acidic soils can raise their pH, limiting aluminium toxicity and increasing phosphorus solubility.
Diagnosis and management
For information about diagnosing phosphorus deficiency and to view more images of phosphorus deficiency symptoms in canola, see DPIRD’s guide to Diagnosing phosphorus deficiency in canola.
Plant tissue testing can be used to confirm suspected phosphorus deficiency. Use whole-shoots and compare paired good and poor plant samples where possible to help diagnosis.
Soil testing before seeding is used to determine soil phosphorus status and “Colwell P” is the standard test used in Western Australia. There are a range of fertiliser decision tools and software applications used by fertiliser companies, agronomists and farmers to interpret soil test results and develop phosphorus fertilisation strategies. These tools consider the PBI of the soil, the soil pH and Colwell P of the soil when determining phosphorus fertiliser requirement which provides a clearer picture than looking at Colwell P alone.
As a guide, approximately 4kg of phosphorus is removed in each tonne of harvested canola seed and this can be used to estimate the amount of phosphorus needed for maintenance of soil phosphorus levels.
A single application of a compound fertiliser is generally drilled at seeding. These fertiliser products usually contain both nitrogen and phosphorus and are made using either MAP (mono-ammonium phosphate) or DAP (di-ammonium phosphate).
Avoid phosphorus toxicity at seeding
Care must be taken not to place high fertiliser levels close to the canola seed as this can cause germination problems or seedling toxicity. This is more likely on very sandy soil types and at high fertiliser rates or on wider row spacing. Banding fertiliser away from the seed can prevent toxicity.
Although phosphorous fertiliser is sometimes top-dressed, this is less effective at supplying young crops because phosphorus is immobile in soils and crop requirement is particularly high during early plant growth.
Remedial action
Phosphorus deficiency usually occurs during early crop development, so it is very important to ensure phosphorus is adequately supplied at sowing. Top dressing phosphorus post seeding is less than half as effective in correcting a deficiency due to its immobility in soils, except on some sandy soils with very low phosphorus buffering index (PBI).
If phosphorus deficiency has been diagnosed by plant tissue testing, it is generally not economic to correct the deficiency during that growing season. Foliar application is ineffective as the amount of phosphorus applied in the spray is small.
Sulfur
Interpreting soil and tissue tests
Yield loss | >25% | 15-25% | 5-15% | <5% |
Sulfur 0-10cm soil test (mg/kg) (in KCI) | <5 | 5-6 | 6-8 | >8 |
Sulfur in whole tops of young plant (%) | <0.2 | 0.2-0.35 | 0.3-0.5 | >0.5 |
- Canola needs more sulfur than wheat
- Mineralisation of soil organic matter can supply significant amounts of sulfate
- Deeper soil testing provides a better picture of the sulfur status of a soil than top soil alone
- Young canola crops sometimes grow through mild deficiency without yield penalty where sulfur levels increase down the soil profile
Sulfur in soils and plants
Canola has a very high requirement for sulfur when compared with cereal and grain-legume crops
Plant available sulfate sulfur comes from applied fertiliser, or the breakdown of soil organic matter. Sulfate is highly soluble so the majority remains available to plants in the soil solution. However a small amount can be adsorbed on soil constituents. On very sandy soils the soluble sulfate is leached from the root-zone early in the growing season leaving young crops sulfur deficient.
Soil type will determine the ability of the soil to supply sulfur to a developing crop. Soil types with high levels of organic carbon can supply significant amounts of plant available sulfur during the growing season through mineralisation. Also, in some WA soil types including Esperance sandplain, sulfur levels can increase down the soil profile because it is retained by iron, aluminium and clay in the subsoil. On these soils, sulfur deficiency is occasionally seen in young canola plants but the roots frequently access sulfur down the soil profile and plants recover without any detrimental effect on crop yield. This phenomenon can reduce the reliability of early plant tissue tests as a predictor of sulfur supply. It also reduces the reliability of soil tests to 0-10cm without deeper soil testing.
The application of nitrogen fertiliser can induce sulfur deficiency on soils where there is not enough plant available sulfur in the soil. Application of nitrogen can stimulate rapid crop growth, increasing demand for sulfur beyond the soils ability to supply. Subsoil constraints that impede root development, such as acidity, sodicity and hardpan can also induce sulfur deficiency.
Estimates for sulfur removal by canola crop can vary, but a survey conducted in Western Australian found each tonne of canola grain removed between 3.2-6.0 kg sulfur, compared with about 1.5-3kg for wheat.
Diagnosis and management
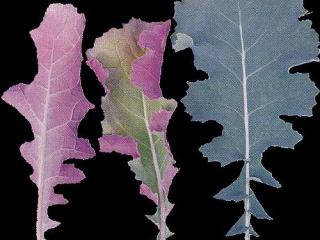
For additional information, and to view images of sulfur deficiency symptoms in canola, see DPIRD's guide to Diagnosing sulfur deficiency in canola.
Plant tissue testing can be used to confirm suspected sulfur deficiency. Use whole-shoots and compare paired good and poor plant samples where possible to help diagnosis. Both soil tests from 0-10cm and early plant tissue tests can over estimate sulfur deficiency and sampling to 30cm provides a better understanding of the distribution of sulfur within the soil profile. Table 3 (above) can help interpret soil or tissue tests through estimating yield loss due to inadequate sulfur.
Soil testing levels less than 7mg S/kg soil in the top 10cm of soil can indicate sulfur deficiency but deeper soil tests can also be helpful determining the sulfur status of soils.
Sulfur nutrition in canola crops is usually managed through the application of sulfur containing compound fertiliser at seeding. These fertilisers supply enough phosphorus for the entire crop, but only a starter amount of nitrogen and sulfur. Additional nitrogen and sulfur are then applied post emergence. Fertilisers with a range of nitrogen: sulfur ratios (N: S ratios)are available as either liquid or granular products. These are achieved by blending ammonium sulfate with urea or adding Sulfur to UAN (urea–ammonium nitrate) products such as Flexi N. Gypsum and ammonium sulfate are the most common sources of applied Sulfur. Gypsum contains approximately 16–18% sulfur, is relatively inexpensive, and unlike ammonium sulfate, it is not acidifying.
Crop constraints that restrict root development such as traffic pans, disease or soil acidity will worsen sulphur deficiency and final yield, even if sulphur is available further down the soil profile. Management strategies that overcome these constraints can help prevent sulfur deficiency. These can include liming, deep ripping and using appropriate rotations and seed treatments to reduce disease development.
Remedial action
Sulfur deficiency can be corrected through the post emergence application of sulfur containing fertilisers. A range of liquid and granular products are available, although foliar sprays generally cannot supply enough sulphur to overcome a severe deficiency. Application of sulfur in the sulfate form using a product such as ammonium sulfate can overcome deficiency rapidly after the fertiliser has moved into the soil profile.
Applying sulfur to crops that are mildly to moderately sulfur deficient does not always increase yield because canola crops sometimes access sulfate down the soil profile, as root systems develop. Transient sulfur deficiency does not usually reduce yield and additional soil testing to 30cm depth combined with farmer knowledge of individual soil types can help predict the ability of a particular soil to supply sulfur to crops.
Potassium
Interpreting soil and tissue tests
Yield loss | >25% | 15-25% | 5-15% | <5% |
Potassium 0-10cm soil test (mg/kg) (Colwell K) | <29 | 29-36 | 36-46 | >46 |
Potassium in whole tops at early seedling stage (%) | <3.8 | 3.9-4.5 | 4.5-5.5 | >5.5 |
Potassium in whole tops at rosette stage (%) | <3 | 3-3.5 | 3.5-4.0 | >4.0 |
- Deeper soil testing provides a better picture of the potassium status of a soil than top soil alone.
- Applying potassium to a mildly deficient crop early in the season may not be economically beneficial as developing root systems can sometimes access additional potassium deeper in the soil profile later in the season.
Potassium in soils and plants
Originally, potassium deficiency in WA was most likely to occur on deep white sands and deep grey sandy duplex soils, but is now also common in the sandy duplex soils and sandy gravels over clays.
Potassium is required for many plant processes including photosynthesis, ionic balance and water use efficiency. Potassium is required in greater amounts than phosphorus and is highly mobile within the plant and if K is in short supply it will move from old to new leaves. Because of this deficiency symptoms are frequently observed on oldest leaves. An adequate supply of potassium provides the plant with increased resistance to disease, frost and drought.
Fertiliser potassium is mobile within the soil so can be prone to leaching beyond the root zone, particularly in the deep sandier soils. Where there is a sub soil constraint thats limits root exploration such as low soil pH or a hard pan, plants will be unable to access leached potassium from deeper in the soil profile.
For canola, the Colwell potassium soil test in the 0-10 cm should be between >46 mg/kg for adequate supply. In sandy duplex soils, soil test potassium levels can fall below 40 mg/kg in the 0-10 cm layer, but potassium levels may rise in the clay subsoil so deeper soil testing is recommended to provide better information about a soil’s ability to supply potassium to canola.
Diagnosis and management
For additional information, and to view images of potassium deficiency symptoms in canola, see DPIRD’s guide to Diagnosing potassium deficiency in canola.
Potassium fertilisers can be drilled at seeding or applied post emergence, if soil test results are below critical levels. The drilled fertiliser products are usually a compound fertiliser containing potassium or a compound fertiliser blended with muriate of potash (MOP, KCl 50% K). Care needs to be taken when drilling potassium fertiliser with the seed as it can decrease seedling emergence under certain conditions.
MOP is usually used for post emergence application; however potassium sulfate (SOP, 42% K) can be used but is more expensive than MOP. Both products are effective at overcoming potassium deficiency.
For soil test levels near the critical Colwell K level, replacement of the quantity of potassium expected to be removed in harvest grain should be considered. This will prevent Colwell soil potassium levels declining to deficient levels over time. Canola grain usually contains about 9kg potassium/tonne.
Remedial action
Top-dressed MOP fertiliser will usually correct the deficiency and muriate of potash is the most widely used product, but the more expensive sulphate of potash can be used. Foliar sprays of potassium generally cannot supply enough potassium to overcome a severe deficiency and can also scorch crops.
Like sulphur, applying potassium to a mildly deficient crop early in the season may not be economically beneficial as developing root systems can sometimes access additional potassium deeper in the soil profile later in the season. Soil testing below 10cm depth and local knowledge of soil types can help predict expected benefit of applying potassium
Nitrogen
Interpreting tissue tests
Yield loss | >25% | 15-25% | 5-15% | <5% |
Nitrogen in whole tops at seedling stage (%) | <2.7 | 2.7-3.0 | 3.0-4.0 | >4.0 |
Nitrogen in whole tops at rosette stage (%) | <4 | 4-4.5 | 4.5-5.1 | >5.1 |
- Mineralisation of soil organic matter can supply significant amounts of nitrate so soil tests alone are a poor predictor of nitrogen supply.
- The peak economic nitrogen application rate is lower than the rate for peak yield.
- Nitrogen supply can affect grain quality characteristics such as oil content
Nitrogen in soils and plants
While nitrogen fertiliser is consistently the largest and most costly input for canola, it represents one of the highest returns on investment. Nitrogen fertiliser generally increases the yield of canola where seasonal conditions allow, although the oil concentration can be reduced. This means the peak economic nitrogen application rate can be lower than the rate for peak yield. High nitrogen canola crops may look fantastic but grain can be downgraded if oil percentage is low. Growers may target a $2 increase in yield for each $1 cost of fertiliser.
Nitrogen for crop growth can be supplied from residue organic nitrogen, soil organic nitrogen and applied fertiliser. Calculate fertiliser needs by assessing soil supply and crop demand and agronomists or fertiliser consultants can help interpret soil tests and formulate nitrogen fertiliser strategies. There are also numerous tools, apps and software products such as SYN, NULogic®and NBroadacre®
Residue organic nitrogen (RON) is generated from the breakdown of legume crop or pastures. Peak supply is in the first year after legume growth, dwindling over the second and third years after legumes. The amount of nitrogen supplied by this source depends on the amount of legume grown (t/ha) and proportion of legume in the pasture, biomass and harvest index of a legume crop and the number of years. This can range from 130kgN/ha for good density legume content pasture, grown in a long season with 400mm GS rainfall, to 260 after an extended pasture phase, or only 20kg/ha for poor density low legume content pasture short season 200mm GS rainfall, or 40kg/ha for a 1t/ha lupin crop.
Soil organic nitrogen (SON) is very closely related to the soil organic carbon (OC) %. Supply of mineralised nitrogen from organic carbon is very stable between years as soil microbes cycle the nitrogen when conditions favour microbial growth. The supply available to plants is reduced by gravel content, which effectively limits the amount of soil available to the plant.
Approximately 50kg/ha per 1% OC with less than 10% gravel, dropping to 30kg/ha for more than 30% gravel but up to 60kg/ha with summer rain. So for 2.5% organic carbon, no gravel summer rain scenario, up to 170kgN/ha but 0.8% OC, no gravel or summer rain, only 40kgN/ha supplied to crops.
Ammonia and nitrates on your soil test show organic nitrogen that has already mineralised for the season, so amounts are generally higher after summer rain. The mineralisation process continues all year and the nitrogen mineralised during the warm wet period in spring is a strong driver of the spring flush.
After adding up the expected supply from RON and SON, we can use fertiliser nitrogen to ensure the total N supply meets the likely N demand of the crop.
N demand= RON supply + SON supply + fertiliser supply
Diagnosis and management
For information about diagnosing nitrogen deficiency and to view more images of deficiency symptoms in canola, see DPIRD’s guide to Diagnosing nitrogen deficiency in canola.
Growers generally apply a starter dose of nitrogen as part of the compound fertiliser drilled at seeding. Post crop emergence, best practice is to apply nitrogen before bolting (approximately 8-10 weeks), as nitrogen uptake is most rapid during stem elongation. However, canola can continue nitrogen uptake past this time, unlike wheat. Later nitrogen top-ups generally have a similar response to applications before bolting; up to early flowering in low and medium rainfall zones and early pod fill in high rainfall zones. This gives farmers a secondary chance to apply nitrogen top-ups in response to a favourable season or as logistics (timing) permit or wet paddock conditions allow.
Remedial action
Post emergence, nitrogen fertiliser is usually top dressed in the form of urea or a blend containing sulphate or, a liquid fertiliser is applied as a foliar spray. Rainfall needs to fall after application before crop roots can access the nitrate although some foliar uptake occurs with foliar spraying. There is a risk of volatilisation loss from urea or nitrate sources of nitrogen. Loss is greatest from dry alkaline soils with dewy conditions, but rarely exceeds 3% per day.
The yield potential for canola is established during stem elongation and the budding stage, so ideally all nitrogen should be applied before this stage of growth (8-10 weeks) although yield responses have been measured from later applications.
Unlike cereals, canola does not ‘hay off’ when too much nitrogen has been applied, but nitrogen reduces oil concentration, particularly with late application.
Micronutrients in canola
Copper
Interpreting soil and tissue tests
Yield loss | >25% | 15-25% | 5-15% | <5% |
Copper 0-10cm soil test (mg/kg) (DTPA) | <0.15 | 0.15-0.25 | 0.25-0.35 | >0.35 |
Copper in seedling whole tops (mg/kg) | <3.0 | 3.0-4.0 | 4.0-5.0 | >5 |
Copper in rosette whole tops (mg/kg) | <2.2 | 2.2-3.0 | 3.0-3.6 | >3.6 |
Copper in youngest emerged leaf (mg/kg) | <2 | 2.0-3.0 | 3.0-4.0 | >4.0 |
- A single application of copper incorporated into soil can prevent deficiency for many years.
- Whole-top plant test provides a rough guide if paired good and poor samples are taken, but this should be confirmed with a youngest emerged leaf test.
Copper in soils and plants
About 8-9 million hectares of Western Australian soils were deficient in copper for agricultural production in their uncleared state. Since clearing, these soils have received widespread application of copper fertiliser at least once and this has allowed large tracts of ‘light land’ in the wheat belt to be used for agricultural production. Copper fertiliser lasts a long time in the soil, so a single application of copper can prevent deficiency for many years and deficiency is now uncommon in canola crops grown in south-west Australia.
Interactions between copper and soil particles (clay, organic matter and iron and aluminium oxides) are complex. The availability of copper to plants would be expected to decrease with liming because in higher pH soils, copper is more strongly bound to soil particles, however, this has not always been observed. Other applied nutrients affect availability of copper to growing crops. For example copper deficiency can be induced in crops if zinc fertilisers are applied, particularly if soil copper levels are marginal. An increase in soil nitrogen either by fertilisation or by mineralisation can also induce copper deficiency in soils with marginal copper concentrations.
The movement of copper in soil is restricted, so it is considered an immobile nutrient. Because of this, applied copper is usually still within in the top 10cm of soil 15 years after the initial application. Therefore top dressing copper fertiliser without cultivation or mixing in the soil profile frequently means that copper is ineffective for crop production. Mixing copper throughout the topsoil makes it more likely that roots will intercept copper mixed through the soil allowing plant uptake.
Copper is adsorbed by plant roots from the soil solution so if it is placed deeper in the profile, where soil is moist for longer, it is more available to the plant resulting in higher plant copper concentrations. The DTPA copper soil test provides a rough guide of the copper status of a soil. Levels of DTPA extractable less than 0.25mg Cu/kg are frequently deficient, with levels of 0.35 adequate for canola production.
Copper is also immobile within plants so deficiency symptoms are usually observed on young leaves and these are the best leaves to sample for plant analysis. The movement of copper in plants was found to parallel nitrogen movement from old to new leaves. Therefore in plants well supplied with nitrogen, where remobilisation of nitrogen is not required from older to younger plant parts, copper also remains in old leaves and new leaves (youngest tissue) may become copper deficient.
Diagnosis and management
For information about diagnosing copper deficiency and to view more images of deficiency symptoms in canola, see DPIRD’s guide to Diagnosing copper deficiency in canola.
A single application of 0.7-1.5kg copper/ha incorporated into copper deficient soils will prevent deficiency of crops for many years as it has a long residual value. As copper is immobile in the soil, incorporation is essential to ensure applied copper does not remain inaccessible to plant roots in the dry top soil.
An alternative is using a compound fertiliser that contains copper, drilled at seeding particularly if DTPA soil test Copper levels are low or plant tissue test from the previous years’ crop indicate low to marginal levels.
Copper foliar sprays can be very effective during the current growing season. However, foliar applications need to be applied before flowering as sufficient copper is required pre-flowering for fertile pollen development. Foliar uptake by leaves is higher in cool and cloudy conditions. A range of products are available for foliar application, with application 125-250g copper/ha, applied in 50-100 litres of water being adequate to correct deficiency. Note that copper sulfate is corrosive and can cause extensive leaf foliage damage, particularly with sprayed a concentration of 2% under sunny conditions.
Copper seed treatment generally does not supply enough copper to meet total plant requirement in the current season. Work with wheat has shown copper seed applications can result in decreased seedling germination under some seasonal conditions, although little research has been done to verify this with canola.
Remedial action
If visual deficiency symptoms or plant tissue tests indicate an in-crop copper deficiency, the only option available to ameliorate deficiency within the current season is applying a copper foliar spray.
Where copper deficiency has occurred, growers should consider a more permanent solution through increasing levels of copper in the soil as outlined in the management section.
Zinc
Interpreting soil and tissue tests
Yield loss | >25% | 15-25% | 5-15% | <5% |
Zinc 0-10cm soil test non alkaline soil (mg/kg) (DTPA) | <0.15 | 0.15-0.25 | 0.25-0.35 | >0.35 |
Zinc 0-10cm soil test alkaline soil (mg/kg) (DTPA) | <0.19 | 0.19-0.31 | 0.31-0.45 | >0.45 |
Whole top zinc at early rosette stage (mg/kg) | <15 | 15-25 | 25-30 | >30 |
Zinc in young mature leaf (mg/kg) | <12 | 12-15 | 15-30 | >30 |
- Canola is moderately susceptible to zinc deficiency; however it is rarely seen in Western Australian crops because of fertiliser usage.
- Tissue tests can provide a more accurate indication of zinc status of canola crops than soil testing.
Zinc in soils and plants
Canola is moderately susceptible to zinc deficiency, however it is rarely seen in Western Australian crops because growers have used fertilisers containing zinc in the past and often use compound fertilisers that contain low levels of zinc.
Zinc is immobile within the soil so having zinc distributed through the soil profile makes it more accessible to developing root systems. Zinc availability is linked to soil pH with deficiency more common on soils with pH above 6.0 as zinc is more strongly bound to soil particles in alkaline soils. Therefore, liming of soils can induce zinc deficiency, although this has been rarely seen in WA.
Whilst soil testing can help understand the zinc status of a particular soil, the commonly used DTPA zinc soil test only provides a rough guide. Tissue tests can provide a more accurate indication of zinc status of canola crops.
Diagnosis and management
For information about diagnosing zinc deficiency and to view more images of deficiency symptoms in canola, see DPIRD’s guide to Diagnosing zinc deficiency in canola.
Many growers sow canola crops with a compound nitrogen and phosphorus fertiliser that contain micronutrients (including zinc) and these fertilisers can provide enough zinc to meet the requirements of that season’s crop.
Seed treatment with zinc is sometimes used to promote early growth (seedling vigour) where root disease is a problem, but the quantity of zinc applied to the seed is less than a plant needs in the current season.
A fertiliser containing zinc needs to be drilled (incorporated) in the soil profile for the zinc to be available to plants. As zinc is immobile in the soil, topdressing is ineffective because it is only available to the plant while the topsoil is wet.
Remedial action
If zinc deficiency occurs in a canola crop, growers can apply a zinc foliar spray but it needs to be applied as soon as deficiency is detected to avoid irreversible damage. As the foliar spray is only effective during the current season, growers are advised to use a drill a fertiliser containing zinc with the next crop.
Manganese
Interpreting tissue tests
Yield loss | >25% | 15-25% | 5-15% | <5% |
Manganese in whole tops of young plant (mg/kg) | <15 | 15-20 | 20-30 | >30 |
- Canola is less susceptible to manganese deficiency than cereals
- No reliable soil test for manganese exists.
Managanese in soils and plants
Manganese deficiency does occur on gravelly soils of the western fringe of the wheat-belt from Moora in the north through Williams and the Boyup Brook-Kojonup area in the south. Similarly, gravelly soils of the Kukerin – Lake Grace area, the Great Southern and the Esperance sand plain are manganese deficient for crops, particularly in drier years. The deficiency is also occasionally observed in crops grown on neutral to alkaline soil types of the Mallee districts, particularly soils north of the Esperance sandplain. Although canola is less susceptible to manganese deficiency than cereals, deficiency does occur in canola crops grown on susceptible soil types, and occasionally on soils that have recently received a heavy application of lime.
As plant available manganese levels can vary rapidly according to soil conditions, a soil test rarely provides an indication of the potential for deficiency. Manganese becomes readily available to plants as soil moisture increases. Manganese in the soil is regularly measured using the DTPA test but research in WA has shown it does not provide a reliable indication of plant available manganese levels. It is therefore important to tissue test canola growing on soils where manganese deficiency is suspected.
Manganese is a nutrient that causes both deficiency and toxicity canola crops. Although manganese toxicity occurs in acid soils in eastern Australia, manganese toxicity has never been confirmed in field grown canola crops of WA.
Diagnosis and management
For information about diagnosing manganese deficiency and to view more images of deficiency symptoms in canola, see DPIRD’s guide to Diagnosing managanese deficiency in canola.
Remedial action
If manganese deficiency is diagnosed in a canola crop, application of a foliar manganese spray is an effective treatment. A foliar application of 4kg manganese sulfate in 50-100 litres of water per hectare at onset of visual symptoms or tissue analyses diagnosis usually corrects the deficiency. However severely deficient patches may require a second application. Other commercially available manganese products should be applied at 1kg manganese per hectare which is the equivalent of 4kg per hectare of manganese sulfate.
Molybdenum
Interpreting tissue tests
Yield loss | >25% | 15-25% | 5-15% | <5% |
Molybdenum in whole tops of young plant (mg/kg) | <0.05 | 0.05-0.1 | 0.1-0.15 | >0.15 |
- Despite many soils being naturally deficient in molybdenum, deficiency has not been diagnosed in canola in Western Australia and there is limited data showing a response to molybdenum fertiliser.
- Molybdenum is less available in acidic soils so liming to maintain topsoil pH above 5.5 is important for maintaining availability.
- There is no available soil test for molybdenum.
Molybdenum in soils and plants
Many slightly acidic to acidic sandplain soils and gravelly sands in WA were molybdenum deficient in their natural state. Application of molybdenum in fertiliser has corrected molybdenum deficiency in many of these soils and deficiency in canola has not been diagnosed in the field in WA. There is however, no reliable soil test for molybdenum.
The risk of deficiency increases on soils that have become more acidic because deficiency is exacerbated at low pH and on soils high in iron and aluminium oxides. However, canola is poorly adapted to acidic soils where molybdenum deficiency would occur so deficiency is difficult to detect in the field.
Maintaining a surface pH above 5.5 through liming is generally accepted to decrease subsoil acidity and this will increase molybdenum availability and ensure no additional molybdenum fertiliser is required.
Diagnosis and management
For more information, and to view images of molybdenum deficiency symptoms in canola, see DPIRD’s guide to Diagnosing molybdenum deficiency in canola.
To confirm a suspected case of molybdenum deficiency, sample whole young plants and take paired good and poor plant samples if possible, although few responses to molybdenum application have been recorded.
Molybdenum deficiency can be prevented by the strategic use of fertilisers containing molybdenum. As molybdenum is immobile in the soil, topdressing is ineffective, as it is only available to the plant while the topsoil is wet. Drilling of molybdenum fertiliser in the soil profile or using a molybdenum seed treatment can ensure availability.
Because molybdenum is less available in acidic soils, liming to maintain topsoil pH above 5.5 is important for maintaining molybdenum availability.
Remedial action
Application of a foliar spray can correct the deficiency and is usually applied as sodium molybdate. Because the foliar spray is effective only in the current season, the following crop may require molybdenum fertiliser. If soil pH is below 4.7, consideration should be given to correction of soil acidity. Molybdenum fertiliser can remain available for several years; however the length of time is dependent on the soil pH. In strongly acid soils molybdenum fertiliser may only remain available for one year.
Boron
Interpreting soil tests
Yield loss | >25% | 15-25% | 5-15% | <5% |
Boron 0-10cm soil test (mg/kg) (in CaCl2) | <0.3 | - | - | 0.3-0.5 |
Yield loss | <5% | 5-15% | 15-25% | >25 |
Boron 10-20cm soil test (mg/kg) (in CaCl2) | 4-18 | 18-25 | 25-30 | >30 |
Boron 20-30cm soil test (mg/kg) (in CaCl2) | 4-18 | 18-25 | 25-30 | >30 |
Boron 30-40cm soil test (mg/kg) (in CaCl2) | 4-18 | 18-25 | 25-30 | >30 |
- Boron can cause both deficiency and toxicity symptoms on different Western Australian soil types, although deficiency is extremely rare.
- Crop varieties may differ in their susceptibility to boron toxicity although we do not have information on the relative susceptibility or tolerance of current canola cultivars.
- There is limited data on critical levels of plant boron for toxicity in canola in WA.
Boron in soils and plants
Boron is an essential nutrient for plant growth and can cause both deficiency and toxicity symptoms on different Western Australian soil types although deficiency is rare. The alkaline, sodic soils most commonly associated with boron toxicity in South Australia are also widespread in the medium to low rainfall areas of WA and this is where boron toxicity of cereals has been reported. Symptoms of boron toxicity are also observed in canola grown on these soil types.
Boron is highly immobile within plants and sampling the whole shoots of plants is unreliable in predicting both boron deficiency and toxicity. As boron is immobile, the youngest leaf or young tissue has been found to be a reliable indicator of boron deficiency in canola.
Soil testing can identify soils with low levels of boron in the surface soil profile that have the potential to develop deficiency in canola. Research has not yet been able to accurately predict which soils will respond to boron application. Similarly, soil testing can identify soils with high levels of boron in the subsoil that have the potential to develop toxicity symptoms in canola. A difficulty for soil testing is that concentrations of boron can vary spatially across a paddock and down the soil profile making diagnosis by soil sampling difficult.
The impact on crop growth depends on the severity of the toxicity, but is also affected by the distribution of boron in the soil. For example, high boron concentration at depth is likely to have less detrimental effect if the plant roots do not have to explore those soil depths for water. If surface soil profiles dry, canola plant roots explore greater soil depth for water and boron uptake increases as a result promoting boron toxicity.
As there have been few responses to boron fertiliser or boron foliar application for canola; particularly for crops grown on low soil boron soils, there is no calibrated soil test for boron deficiency. Soil testing may be useful on deep acidic sands with low clay and low organic carbon but on duplex soils subsoil boron levels should also be determined. In recent years, research on 30 canola crops grown on soils where boron deficiency would be anticipated have shown no response to boron fertiliser or sprays despite soil tests having very low boron levels (around 0.3 mg/kg boron).
Elevated boron levels can also reduce calcium absorption in canola which may induce calcium deficiency/tipple topple. For more information about diagnosing calcium deficiency and to view images of deficiency symptoms in canola, see DPIRD’s guide to Diagnosing calcium deficiency in canola.
Diagnosis and management
For more information about diagnosing boron deficiency and to view more images of deficiency symptoms in canola, see DPIRD’s guide to Diagnosing boron deficiency in canola.
As boron is less available in acidic soils, liming to maintain topsoil pH can increase soil boron availability.
Management options for boron toxicity are limited to using tolerant crops and genotypes. We do not have information on the relative susceptibility or tolerance of current canola cultivars to boron toxicity.
Remedial action
Boron deficiency can be corrected by soil or foliar applications of boron. Soil applications generally last longer but can be leached from acidic, sandy soils. Boron, supplied as borax is very toxic to seedling germination and seed yield can greatly be reduced if higher rates than necessary are applied.
There are no in crop remedial actions for boron toxicity. For information about remedial actions for boron deficiency, see management strategies section above.
Other soil constraints affecting canola nutrition
Canola's ability to access nutrients can also be affected by the physical and chemical properties of the soil. Soil constraints which restrict root growth can limited the plants ability to access nutrients and soil chemical properties such as pH can affect the availability of some nutrients.
Critical levels for common soil constraints are presented here to provide some interpretation of soil tests specifically for canola crops.
As with macro and micro nutrients, a ‘traffic light’ format has been used with red indicating greater than 25% yield loss is likely, orange indicating between 15-25% yield loss, yellow between 5-15% yield loss and green less than 5% yield loss. Canola growers can use this information to easily determine the urgency of addressing a particular soil constraint.
These critical values can be used as a guide to the approximate levels of yield loss expected. They are necessarily simplified and generalised and are not intended or able to replace professional assessment and recommendations.
Soil pH and aluminium toxicity
Acidic soil types are widespread in Western Australia's grain growing region and soil acidity is a common constraint to canola yield which is more sensitive than wheat. Low soil pH can reduce the availability of certain nutrients such as phosphorus and can increase concentrations of toxic elements such as aluminium in the soil solution. Canola is sensitive to high aluminium levels in the subsoil and aluminium toxicity is the major problem associated with soil acidity in Western Australia.
Alkalinity can also reduce seed yield of canola crops on certain soil types.
For more information, and to view images of the symptoms of soil acidity in canola, see DPIRD’s guide to Diagnosing soil acidity in crops.
For general information about soil pH, it's impact on crop production and management options, refer to Soil acidity in Western Australia.
Interpreting acidic soil pH tests for canola
Yield loss | >25% | 15-25% | 5-15% | <5% |
Acid soil (pH) 0-10cm soil test (in CaCl2) | <4.5 | 4.5-5.0 | 5.0-5.5 | 5.5-7.5 |
Acid soil (pH) 10-20cm soil test (in CaCl2) | <4.5 | 4.5-5.0 | 5.0-5.5 | 5.5-7.5 |
Acid soil (pH) 20-30cm soil test (in CaCl2) | <4.5 | 4.5-5.0 | 5.0-5.5 | 5.5-7.5 |
Interpreting alkaline soil pH tests for canola
Yield loss | <5% | 5-15% | 15-25% | >25 |
Alkaline soil (pH) 0-10cm soil test (in CaCl2) | 5.5-7.5 | 7.5-8.0 | 8.0-8.5 | >8.5 |
Alkaline soil (pH)10-20cm soil test (in CaCl2) | 5.5-7.5 | 7.5-8.0 | 8.0-8.5 | >8.5 |
Alkaline soil (pH) 20-30cm soil test (in CaCl2) | 5.5-7.5 | 7.5-8.0 | 8.0-8.5 | >8.5 |
Interpreting soil aluminium tests for canola
Yield loss | >25% | 15-25% | 5-15% | <5% |
Soil aluminium 10-20cm soil test (mg/kg) | >5 | 4.0-5.0 | 2.0-4.0 | <2.0 |
Soil aluminium 10-20cm soil test (mg/kg) | >5 | 4.0-5.0 | 2.0-4.0 | <2.0 |
Soil sodicity
Dispersive or sodic soils are common in the south-west agricultural area of Western Australia where they occur mainly as duplex or gradational profiles on broad, flat landscapes with poor drainage. They are structurally unstable when wet and lack structure when dry. Soil aggregates collapse when the soil gets wet because the individual clay particles disperse into solution. This collapse of structure causes the soil to slump, lose porosity and become denser thus restricting crop root growth. This leads to problems including waterlogging, reduced plant growth, increased erosion risk, and poor trafficability when wet. These soils are difficult to manage, and can have multiple constraints to crop and pasture growth.
Soil testing that measures the exchangeable sodium percentage in soil solution is used to measure soil sodicity. Table 15 presents estimates of yield loss due to sodicity for 0-10cm soil tests. Sodicity below 10cm can also reduce yields, but impacts are likely to be less than for 0-10cm.
For more information, and to view images of the symptoms of poor seedbed soil structure, see DPIRD’s guide to Diagnosing poor seedbed soil structure.
For more information, and to view images of the symptoms of waterlogging in canola, see DPIRD’s guide to Diagnosing waterlogging in canola.
For more general information about dispersive and sodic soils in Western Australia including management options refer to Dispersive and sodic soils.
Interpreting soil sodicity tests for canola
Yield loss | >25% | 15-25% | 5-15% | <5% |
Soil sodicity - exchangeable sodium (%) | >15 | 6-15 | 2-6 | <2 |
Soil compaction
Livestock and light machinery can compact surface soil, and agricultural machinery or natural soil characteristics and conditions can lead to subsurface compaction. Some soils have greater capacity to resist compaction or to self-repair following compaction. Compacted sub-surface soil restricts crop root growth.
Soil compaction is not assessed as a part of standard soil testing, however a hand held penetrometer can be used to locate the depth and severity of a hard pan. Assessment must be done in moist soil conditions.
For more information, and to view images of the symptoms of soil compaction, see DPIRD’s guide to Diagnosing sandplain traffic pan
For more general information about soil compaction in Western Australia including management options, refer to Soil compaction.
Interpreting soil strength tests (compaction) for canola
Yield loss | >25% | 15-25% | 5-15% | <5% |
Sandy soil - penetration resistance (MPa) | >2.2 | 1.9-2.2 | 1.6-1.9 | <1.6 |
Duplex soil - penetration resistance (MPa) | >2.6 | 2.2-2.6 | 1.7-2.2 | <1.7 |
Clay soil - penetration resistance (MPa) | >4.5 | 3.8-4.5 | 3.0-3.8 | <3.0 |
Soil salinity
Dryland salinity is a major form of land degradation. More than one million hectares of broadacre farmland in Western Australia is estimated to be currently affected by dryland salinity. Through activities such as groundwater and soil analysis, landholders can confidently assess salinity risks and implement appropriate management responses.
Canola has a similar level of tolerance as wheat to soil salinity which is substantially less tolerant than barley. Crop growth is affected by the concentration of salt in the soil solution rather than just in the soil. When there is less water in the topsoil, the soil solution will be more concentrated, which may cause a higher yield loss even with a low salinity reading. As such, it is hard to assign salinity limits to yield loss categories. Instead, the traffic lights shown below correspond to severe, very high, high and low risk of yield decline due to salinity.
For more information, and to view images of the symptoms of soil acidity in canola, see DPIRD’s guide to Diagnosing soil salinity in canola.
For more general information about soil salinity in Western Australia, refer to Soil salinity.
Salinity is measured as electrical conductivity (EC) and is expressed in different units by different companies. This is based on a 1:5 soil:water solution. In Table 18, the data is expressed in millisiemens per metre (mS/m). If the units of your data are different to mS/m, you will need to convert it by multiplying with the conversion factors in Table 17:
Unit | Conversion factor | millisiemens per metre |
---|---|---|
milligrams per litre (mg/L) | x 0.18 | = ms/m |
parts per million (ppm) | x 0.18 | = ms/m |
micro siemens per centimetre (µS/cm) | x 0.1 | = ms/m |
decisiemens per metre (dS/m) | x 100 | = ms/m |
Interpreting soil salinity tests for canola
Yield loss | Extreme risk of yield loss | High risk of yield loss | Moderate risk of yield loss | Low risk of yield loss |
Soil salinity - EC 1:5 (mS/m) | >100 | 75-100 | 50-75 | <50 |